In 1924 an Indian physicist called Satyendra Nath Bose wrote to Albert Einstein saying he had solved a problem in quantum physics that had stumped the great man. One century on, Robert P Crease and Gino Elia explain how the correspondence led to the notion of Bose–Einstein condensation and why it revealed the power of diverse thinking
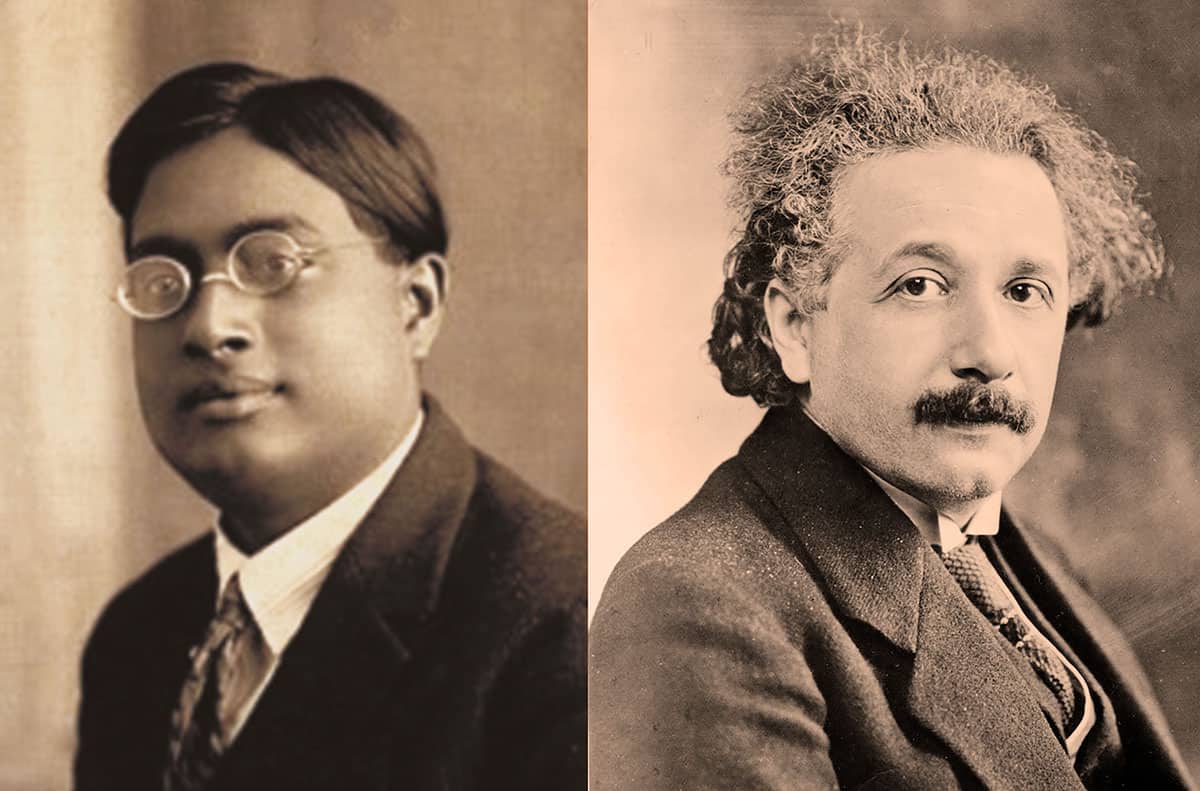
One day in June 1924, Albert Einstein received a letter written by a professor in India. The author admitted he was a “complete stranger” but said he was sending Einstein an accompanying article for his “perusal and opinion”. Just five pages long, the article claimed to address a flaw in quantum theory that Einstein had struggled unsuccessfully with for several years.
Einstein, who was then at the University of Berlin, immediately realized that the author – Satyendra Nath Bose – had solved the problem that had defeated him. It concerned a fully satisfactory derivation of Planck’s law, which describes the spectrum of radiation from a black body. First derived by Max Planck in 1900, the law showed that the radiation does not rise to infinity at ever-shorter wavelengths as classical physics suggests, but instead peaks before falling back.
Einstein quickly developed Bose’s approach further in his own work and, as a result of their collaboration, the pair predicted the existence of a new phenomenon, dubbed “Bose–Einstein condensation”. Anticipated to occur at very low temperatures, it would involve all particles in a system occupying the same lowest quantum state. This new collective state of matter was experimentally detected for the first time in 1995, leading to Eric Cornell, Wolfgang Ketterle and Carl Wieman winning the Nobel Prize for Physics six years later.
The Bose–Einstein exchange may have been brief, but it is one of the great correspondences in the history of physics. Writing in the 2020 book The Making of Modern Physics in Colonial India, the historian and philosopher of science Somaditya Banerjee, who is now at Austin Peay State University in Clarksville, Tennessee, says their collaboration illustrated the growing importance of international joint efforts in science. Or, as Banerjee puts it, their work revealed the “transnational nature of the quantum”.
Marginalized inspiration
Bose grew up politically and scientifically marginalized. He was born on 1 January 1894 in Kolkata (then Calcutta) in the Indian state of Bengal, which was under British occupation, to a family that was part of a cultural and educational movement called the “Bengal renaissance”. Its members had an ambivalent relationship with European culture, partly rejecting and partly embracing it.
Bose and Saha felt alienated from and antagonistic towards the British colonizers, and did not want to serve them by contributing to fields with possible practical applications
In 1905, when Bose was 11, the British occupiers – alarmed by growing rebelliousness in Bengal – split the state into two. Part of the reason Bose went into academia, according to Banerjee, may have been a nationalist urge to avoid being conscripted into the colonial bureaucracy, which was the fate of many middle-class Bengalis.
Bose instead attended Presidency College with his friend (and future astrophysicist) Meghnad Saha, who had been expelled from his school for his involvement in the “Swadeshi movement”. Seeking to curb the use of foreign goods and rely instead on domestic products, the movement was part of the push for Indian independence and stood against the proposed partition of Bengal.
Bose and Saha felt alienated from and antagonistic towards the British colonizers, and – like many of their peers – did not want to serve them by contributing to fields with possible practical applications, such as chemistry or applied physics. The pair were instead attracted by mathematics and theoretical physics – and in particular by the new-fangled quantum theory that German physicists were pioneering.
According to Banerjee, Bose saw his work as “an intellectual escape from the inequities and asymmetries of power relationships” in occupied Bengal. “It is thus no accident,” he writes, “that the emerging Indian physicists particularly excelled in quantum physics.” As a result of their familiarity with German work, Bose and Saha were highly influenced by photon theory, which implied discontinuities in light. British physicists, in contrast, were more impressed by the continuous nature of light dictated by Maxwell’s equations.
Bose and Saha both went on to become physics instructors at the University of Calcutta. But due to Bengal’s isolation and the effects of the First World War, they found it hard to follow the most recent developments in Europe. One of the few periodicals that was regularly available in the Presidency library was Philosophical Magazine, in which Bose and Saha read one of Niels Bohr’s seminal papers on atomic structure, published in 1913 (Phil Mag. 26 1).
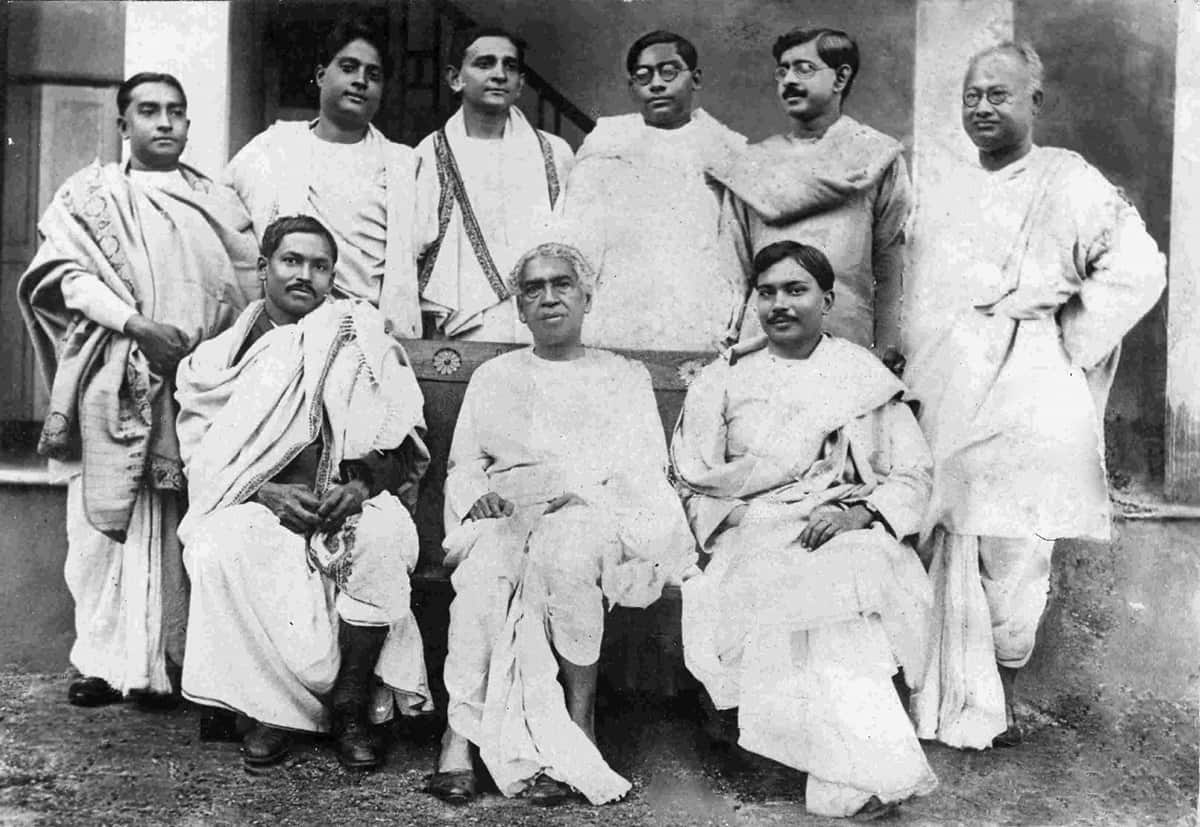
In Calcutta, they were also fortunate to befriend Paul Johannes Brühl, a visiting botanist from Germany, who had brought with him books and journals on thermodynamics, quantum theory, relativity and other popular physics topics. In 1919, after Einstein had shot to fame following the apparent confirmation of general relativity, Bose and Saha managed to get copies of the basic papers in German and French. Bose was fluent in both languages, as well as English, and so he and Saha translated and published the papers in book form as The Principle of Relativity (University of Calcutta, 1920). It was the first English-language collection of papers on the topic from Einstein and others.
Then, in 1921, Bose was awarded a professorship at the recently established Dacca (now Dhaka) University and charged with developing its physics department. Two years later, rather suddenly, severe budget cuts ended the plan to expand the department, and Bose even had to fight to keep his job. In 1923, therefore, Bose found himself in an unresolved professional state, at a stressful political time in an occupied land.
The Einstein connection
Despite his problems, the 30-year-old continued carrying out research. Later that year, he pondered a disturbing fact: the derivation of Planck’s law was logically unsound as it mixed classical and quantum concepts. Bose decided to ignore classical theory and derive the law instead by considering the motions of a gas of discrete photons. He outlined his thoughts in autumn 1923 in his now seminal paper entitled “Planck’s law and the light-quantum hypothesis”, a version of which he would shortly send Einstein.
Planck’s law, the paper began, is the starting point for quantum theory. But one crucial formula in deriving it relies on a classical assumption about available degrees of freedom. “This is an unsatisfactory feature in all derivations,” Bose wrote. While admitting that Einstein’s own attempt to derive the law free from classical assumptions was “remarkably elegant”, Bose did not feel it was “sufficiently justified from a logical point of view”.
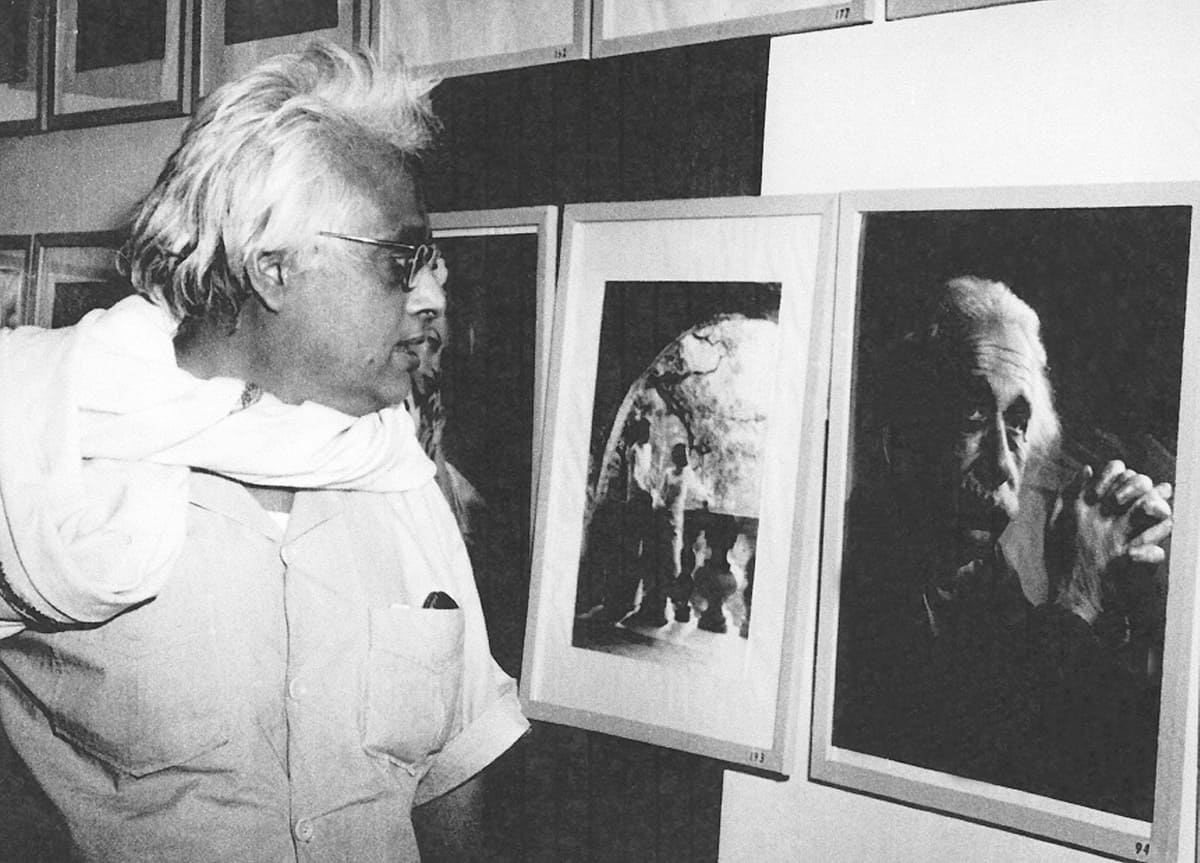
Bose boldly continued: “In the following I shall sketch the method briefly.” Three pages of rigorous derivations follow, culminating in an equation describing the distribution of energy in the radiation from a black body. This equation, Bose proclaimed, was “the same as Planck’s formula”.
In a recent paper on arXiv (arxiv.org/abs/2308.01909), the physicist Partha Ghose, who was one of Bose’s last PhD students, says that Bose’s method hinted at – but was not explicit about – the indistinguishability of those individual photons. Bose instead defined a volume for photons as a space composed of states – which he called cells – with the total number of cells equalling the number of ways in which the photons can be arranged. As the gas of photons has a fixed density, rearranging individual photons doesn’t produce new cells, implying the photons themselves cannot be told apart; you can not “tag” them to follow them around.
Bose sent the paper to Philosophical Magazine – which he knew was available to Indian physicists – around the beginning of 1924, but never heard back. Disappointed, but convinced of its soundness, he sent it, or a slightly revised version, to Einstein, who received it on 4 June 1924.
“An important step forward”
Einstein was primed. He knew the inconsistency of using a classical assumption to derive a quantum law and had already made several unsuccessful attempts to remove it. Bose’s derivation was sound, Einstein realized.
Einstein gleaned more significance in Bose’s work than Bose himself, for he spotted an unexploited analogy
On 2 July of that year, Einstein responded with a hand-written postcard to Bose calling the paper “an important step forward”. Einstein then translated the paper himself and sent it to Zeitschrift für Physik. With Einstein’s endorsement, Bose’s paper was accepted, and it was duly published in the journal in August 1924 (26 178).
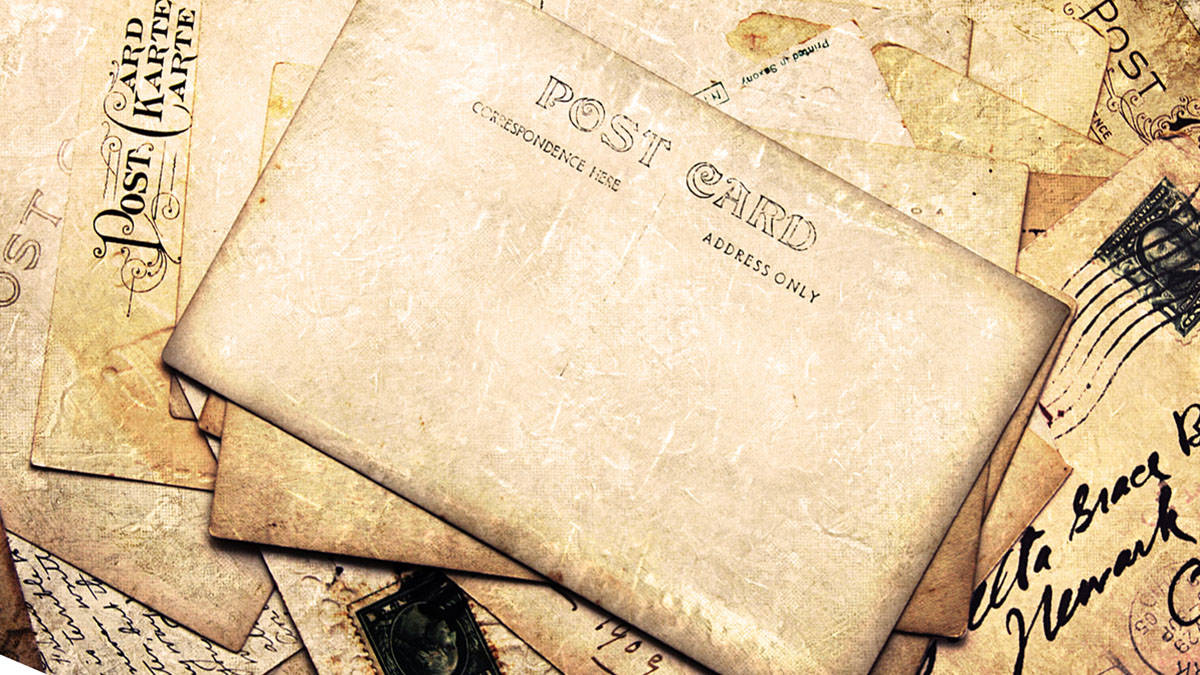
Einstein gleaned more significance in Bose’s work than Bose himself, for he spotted an unexploited analogy. Essentially, Bose had treated the photons as statistically dependent, implying the possibility of wave interference. What Einstein realized was that this didn’t have to apply only to photons but could apply to other particles as well. In fact, as we now know, interference is true only of particles with integer values of spin, or what Paul Dirac, two decades later, dubbed “bosons”. These contrast with “fermions”, whose spin comes in odd half-integer values.
Shortly after receiving Bose’s note, Einstein wrote a German-language paper entitled “Quantentheorie des einatomigen idealen Gases” (or “Quantum theory of the monatomic ideal gas”). Published in the Proceedings of the Prussian Academy of Sciences in January 1925, it described what Einstein called “a far-reaching formal relationship between radiation and gas”. The paper essentially showed that at temperatures near absolute zero, the entropy of a system disappears altogether, and all particles drop to the same state or cell. Within each cell, the entropy of the molecular distribution “expresses indirectly a certain hypothesis concerning a mutual influence of the molecules which is of a quite mysterious nature”.
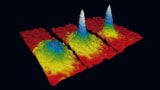
Coldest: how a letter to Einstein and advances in laser-cooling technology led physicists to new quantum states of matter
Einstein attributed this influence to the interference of particles. At low temperatures, he predicted, the wavelike characteristics of gases like hydrogen and helium would become more pronounced, to the point where viscosity would rapidly decrease – a phenomenon now called “superfluidity”. By insisting on treating the analogy between radiation and gases as exact, Einstein had built on Bose’s work to end up predicting an unknown state of matter.
Thanks to Einstein’s attention to Bose’s work, the latter received a two-year sabbatical to study in Europe. Bose travelled first to Paris in the autumn of 1924, where he wrote two further letters to Einstein. The following year he went to Berlin where he was finally able to speak to Einstein in person in early 1926. But the pair never got around to collaborating further. Einstein objected to Bose’s probability formula for the states of particles in a radiation field at thermal equilibrium, and Bose, involved with other things, did not return to this particular question. Their June 1924 exchange, however brief, remained the most productive part of their correspondence.
How hot the vacuum
Eventually, some 70 years later, this new state of matter, now called Bose–Einstein condensation (BEC), was experimentally demonstrated at two labs in the US in 1995. That, too, was the outcome of a long series of developments, for in 1924, BEC was just a limit case of quantum gases, seen as becoming possible only near absolute zero. It seemed unreachable; even raw vacuum is too hot for BEC.
A turning point was the invention, in 1975, of laser cooling. By tuning the frequency of laser light just below that of target atoms, physicists could fire photons at atoms moving in the opposite direction. Thanks to the Doppler effect, the atoms could then be tricked into absorbing the photons while pushing them in the opposite direction of the laser, reducing their velocity and causing them to cool.
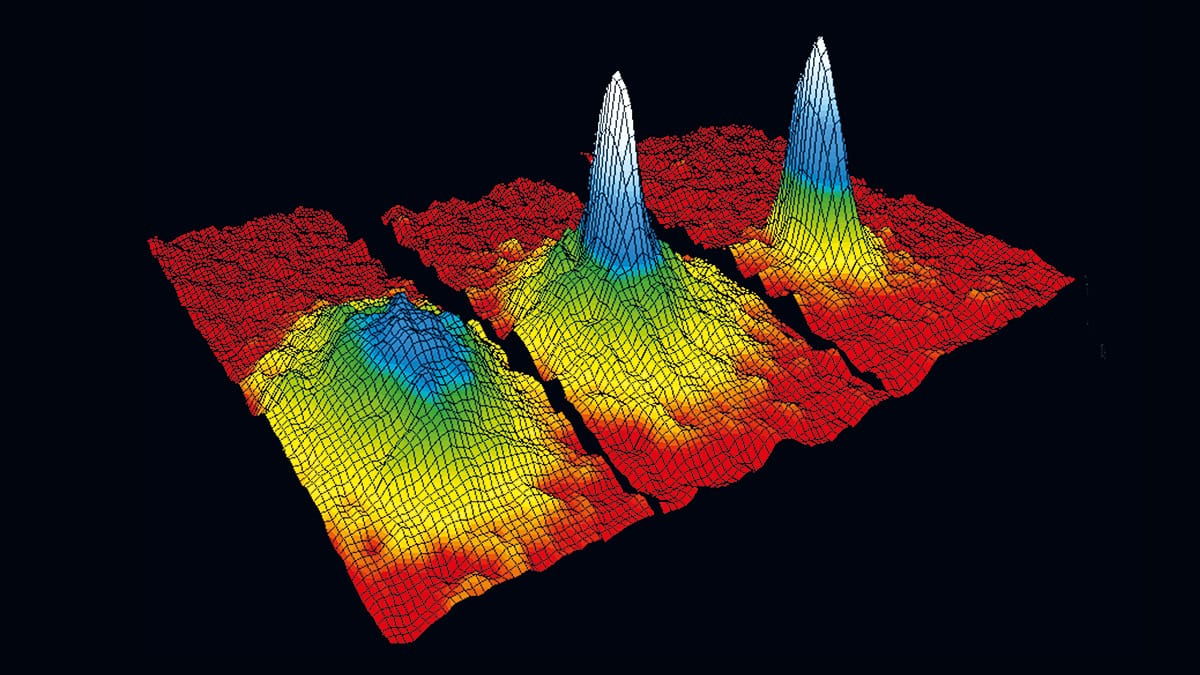
A year later, a group of physicists showed that isotopes of hydrogen could be cooled to replicate BEC. In 1989, Cornell and Wieman settled on rubidium atoms because they would group faster than hydrogen. Sometimes referred to as “super atoms”, BEC occurs when the wave packets of individual particles overlap and become fully indistinguishable at low temperatures.
Wieman and Cornell described BEC as a “quantum identity crisis” that happens when the atoms clump together in the lowest possible state of the system. The intrigue of creating a giant wave packet is that BEC gives us a window to witness quantum behaviours on a macroscopic level.
The critical point
“The correspondence between Bose and Einstein,” Banerjee wrote in The Making of Modern Physics in Colonial India, “is a special moment in the history of science”. Bose did not come from out of the blue to contribute a piece to a growing jigsaw puzzle. By virtue of his working far from Europe in a colonized land, Banerjee argues, Bose was uniquely poised to facilitate change in Western thinking about quantum theory.
Bose’s work was not the first time that non-Western scientists had contributed key insights to European science. But his collaboration with Einstein illustrates a deeper point – namely how regional differences can give different senses of what’s important and what is not. As Banerjee puts it, Bose’s contribution illustrates the “locally rooted cosmopolitanism” of science.
Diversity in worldviews, not cultural conformity, holds the most powerful promise for progress in physics.
Robert P Crease (click link below for full bio) is a professor in the Department of Philosophy, Stony Brook University, US, where Gino Elia is a PhD student