In the search for a cosmological model that perfectly explains our universe, most astronomers invoke the notion of dark matter. But what if they should instead modify the age-old laws of gravity? In the first of a three-part series, Keith Cooper explores the struggles and successes of modified gravity in explaining phenomena at varying galactic scales, as well as matching observations from the cosmic microwave background
Imagine if, in one fell swoop, with one small tweak to the laws of gravity, you could wave away the need for all the dark matter in the universe. You’d rid yourself of a pesky particle that is only inferred to exist and has so far defied discovery. Instead, you would replace it with an elegant theory that modifies the fundamental work of Isaac Newton and Albert Einstein.
At least that’s the dream of modified Newtonian dynamics, or MOND. Developed by Israeli physicist Mordehai Milgrom and Mexican-born American-Israeli theorist Jacob Bekenstein in the early 1980s, it was their antidote to the popular “dark matter” paradigm. To them, dark matter was an unnecessary and clumsy bolt-on to cosmology that, if real, means that 80% of the matter in the cosmos is invisible.
In the 40 years since it was devised, MOND’s achievements continue to be overshadowed by cosmology’s love affair with dark matter. MOND has also struggled to explain phenomena at scales larger and smaller than individual galaxies. So is MOND something we should be taking seriously after all?
Curious curves
Our story starts in the late 1960s, and into the 1970s, US astronomers Vera Rubin and Kent Ford realized that stars on the outskirts of galaxies were orbiting just as fast as stars close to the centre, in apparent defiance of Johannes Kepler’s laws of orbital motion. They illustrated this in the galaxies’ rotation curves, essentially just a graph of orbital velocity versus radius from the centre. Rather than showing a negative slope, the graphs were a flat line. Somewhere, there was some extra gravity pulling those outer stars around.
Dark matter – an unseen form of matter so abundant that it would be the dominant gravitational force in the universe – was the popular solution. Today, the concept of dark matter is intimately entwined in our standard model of cosmology and is inherent in our understanding of how structure in the universe forms.
1 Disc proof
(a) NGC 253 is a bright spiral, or disc, galaxy about 13 million light-years from Earth in the southern constellation of the Sculptor. (b) Stacy McGaugh from Case Western Reserve University in the US and colleagues derived a universal law governing the rotation of disc galaxies. The law indicates that the rotation of such galaxies is specified by the visible matter it contains, even if the galaxy is mostly composed of dark matter.
The picture that dark matter forms is neat, but not quite neat enough for a small community of physicists and astronomers who have shunned dark-matter cosmology and adopted MOND instead. In fact, they have abundant evidence for their case. In 2016 Stacy McGaugh of Case Western Reserve University measured the rotation curves of 153 galaxies (Phys. Rev. Lett. 117 201101) and found, with an unprecedented accuracy, that their rotation curves are explained by MOND, without the need to resort to a halo of dark matter around each galaxy. In doing so, he justified Milgrom’s prediction.
“I would assert that MOND explains these things better than dark matter, and the reason for that is its predictive power,” says McGaugh – a former dark-matter researcher who is now a MOND advocate, following an epiphany that saw him switching sides. He is referring to the fact that if you know the visible mass (all its stars and gases) of a galaxy, then by applying MOND you can calculate what the rotation velocities are going to be. In the dark-matter paradigm, you can’t predict the velocities based on the presence of dark matter. Instead, you have to measure the galaxy’s rotation curve to infer how much dark matter is present. McGaugh argues that’s circular reasoning, and not proof of dark matter.
How to modify gravity
Modifying the laws of gravity might be anathema to many physicists – such is the power of Newton and Einstein – but it’s not such an outlandish thing to do. After all, we live in a mysterious universe, filled with scientific conundrums. What is the dark energy responsible for the acceleration of the universe’s expansion? Why is there a tension in different measurements of the expansion rate of the universe? How are galaxies forming so quickly in the early universe, as witnessed by the Hubble and James Webb space telescopes? Researchers are increasingly looking at modified gravity theories to provide the answers, but not all modified gravity models are equal.
What every theory of modified gravity, including MOND, must do is explain why it remains hidden from us on everyday scales, only kicking into action under certain conditions
Tessa Baker, a cosmologist and modified gravity guru at the University of Portsmouth in the UK, has built her career on testing the laws of gravity and searching for modifications, in her case to try to explain dark energy. “MOND, which is one example of a modified gravity theory, is unusual in that it is a theory that tries to replace dark matter,” explains Baker. “The majority of theories of modified gravity do not do that.”
What every theory of modified gravity, including MOND, must do is explain why it remains hidden from us on everyday scales, only kicking into action under certain conditions. Physicists call the point at which this transition occurs as “screening”, and it’s all a problem of scale.
“The tricky part is, how do you hide the modification on scales where we know general relativity works very well?” asks Baker. The obvious place to start might be considering whether gravity varies on a distance scale, so in our solar system gravity fades with the inverse-square rule, but on the scale of galaxy clusters it decreases at a different rate. “This categorically does not work,” says McGaugh, adding that there are other scales that do work.
For example, one theory of modified gravity that Baker works with – known as f(R) gravity – generalizes Einstein’s general theory of relativity. Under f(R), gravity switches on the dark-energy effect in areas of space where the density of matter becomes low enough, such as in cosmic voids. For MOND, the scale of the screening mechanism is acceleration. Below a characteristic gravitational acceleration referred to as a0 – which is about 0.1 nanometres per second squared – gravity operates differently.
Rather than following the inverse-square rule, at accelerations below a0 gravity drops off more slowly, by the inverse of distance. So something orbiting at four times the distance would feel a quarter of the gravity, not a 16th. The low gravitational accelerations necessary for this are exactly those experienced by stars on the outskirts of galaxies. “So MOND switches on those modifications at low accelerations in the same way that f(R) gravity switches on its modifications at low densities,” explains Baker.
Conflict and controversy
MOND excels for individual galaxies, but depending on whom you speak to, it’s perhaps not doing so well in other environments. And one failure in particular has already turned one of MOND’s staunchest supporters against the theory.
An ideal laboratory in which to test MOND is one where dark matter would not be expected to be present in any great amounts, meaning any gravitational anomalies should just come from the laws of gravity themselves. Wide binary star systems are one such environment consisting of pairs of stars that are 500 AU or more apart (where one astronomical unit or AU is the mean distance between Earth and the Sun). At such huge separations, the gravitational field felt by each star is weak.
Thanks to the European Space Agency’s Gaia astrometric space mission, teams of MOND researchers have now been able to measure the motions of wide binaries in search of evidence of MOND. The results have been controversial and conflicting, in terms of the survival of MOND as a valid theory.
One team, led by Kyu-Hyun Chae of Sejong University in Seoul, carried out an exhaustive analysis of 26,500 wide binaries and found orbital motions that matched the predictions of MOND (ApJ 952 128). This was supported by earlier work from Xavier Hernandez of the Universidad Nacional Autónoma de México, who hailed how “exciting” Chae’s result were. But not everyone is convinced.
2 Testing ground
(left) Wide binary star systems such as this one should be an ideal test for MOND because the effect of dark matter should be minimal, so gravitational effects should come solely from the laws of gravity. (right) Kyu-Hyun Chae of Sejong University in Seoul tested this by analysing observations of more than 20,000 wide binary systems. He found a consistent gravitational anomaly (a boost factor of 1.4) at accelerations lower than 0.1 nm/s2. This agrees with the original MOND theory.
At the University of St Andrews in the UK, Indranil Banik was working on his own six-year project to measure MOND in wide binaries. He had published his plans ahead of taking his measurements, making sure to take time to talk to other experts and get feedback, fine-tuning his method so that everyone could be in agreement. Banik fully expected his results to show that MOND was real. “I obviously expected the MOND scenario to work,” he says. “So it was indeed a very major surprise when it didn’t.”
In a paper published in late 2023 Banik found no deviation from standard Newtonian gravity at all (Monthly Notices of the Royal Astronomical Society 10.1093/mnras/stad3393). The results were such a hammer blow to him that it shook Banik’s world, and he publicly declared that MOND was wrong – which caught him some flak. Why, though, should his results be so different to Chae and Hernandez? “Certainly, they still argue there is something there,” says Banik. However, he is sceptical of their results, citing differences in how they dealt with uncertainties in their measurements.
These points of contention are highly technical, so it’s perhaps not a total surprise that different interpretations have been arrived at. Indeed, for outsiders it is difficult to know who is correct and who isn’t. “It’s very hard to know how to judge this,” admits McGaugh. “I don’t even feel entirely qualified to judge on those scales, and I’m way more qualified than most people!”
It’s not just wide binaries where Banik sees MOND failing. He also cites the case of our own solar system. One of the central tenets of MOND is the phenomenon of the “external field effect”, whereby the overall gravitational field of the Milky Way galaxy is able to imprint itself on smaller systems, such as our solar system. We should see this imprint, particularly on the orbits of the outer planets. Searching for this effect through radio-tracking data from NASA’s Cassini spacecraft, which orbited Saturn between 2004 and 2017, has found no evidence for the external field effect on Saturn’s orbit.
“People are starting to realize that there is no way to reconcile MOND with the non-detection of effects in the Cassini data and that MOND will not work on scales below a light-year,” says Banik. If Banik is correct, then it leaves MOND in a very bad place – but it’s not the only battlefield where MOND’s war against dark matter is being fought.
Cluster conundrums
In 2006 NASA released a spectacular image of two colliding clusters of galaxies, referred to in their combined form as the Bullet Cluster. The Hubble Space Telescope provided high-resolution views of the whereabouts of the galaxies, while X-ray observations of the hot gas between those galaxies came from the Chandra X-ray Observatory. Based on the locations of the galaxies and gas, as well as the degree of gravitational lensing as matter in the cluster bent space, scientists were able to calculate the location of the dark matter in the cluster.
“It was claimed that the Bullet Cluster confirmed the existence of dark matter, which has been used to argue strongly against MOND,” says Pavel Kroupa, an astrophysicist at the University of Bonn. “Well, it turns out that the situation is exactly the opposite.”
Kroupa is ferocious in his enthusiasm for MOND, and has set his sights on exploring it on the largest scales of structure possible – large-scale galaxy clusters. In his crosshairs is nothing less than the standard model of cosmology, known colloquially as “lambda-CDM” or ΛCDM (Λ refers to the cosmological constant, or the dark energy component of the universe, and CDM is cold dark matter).
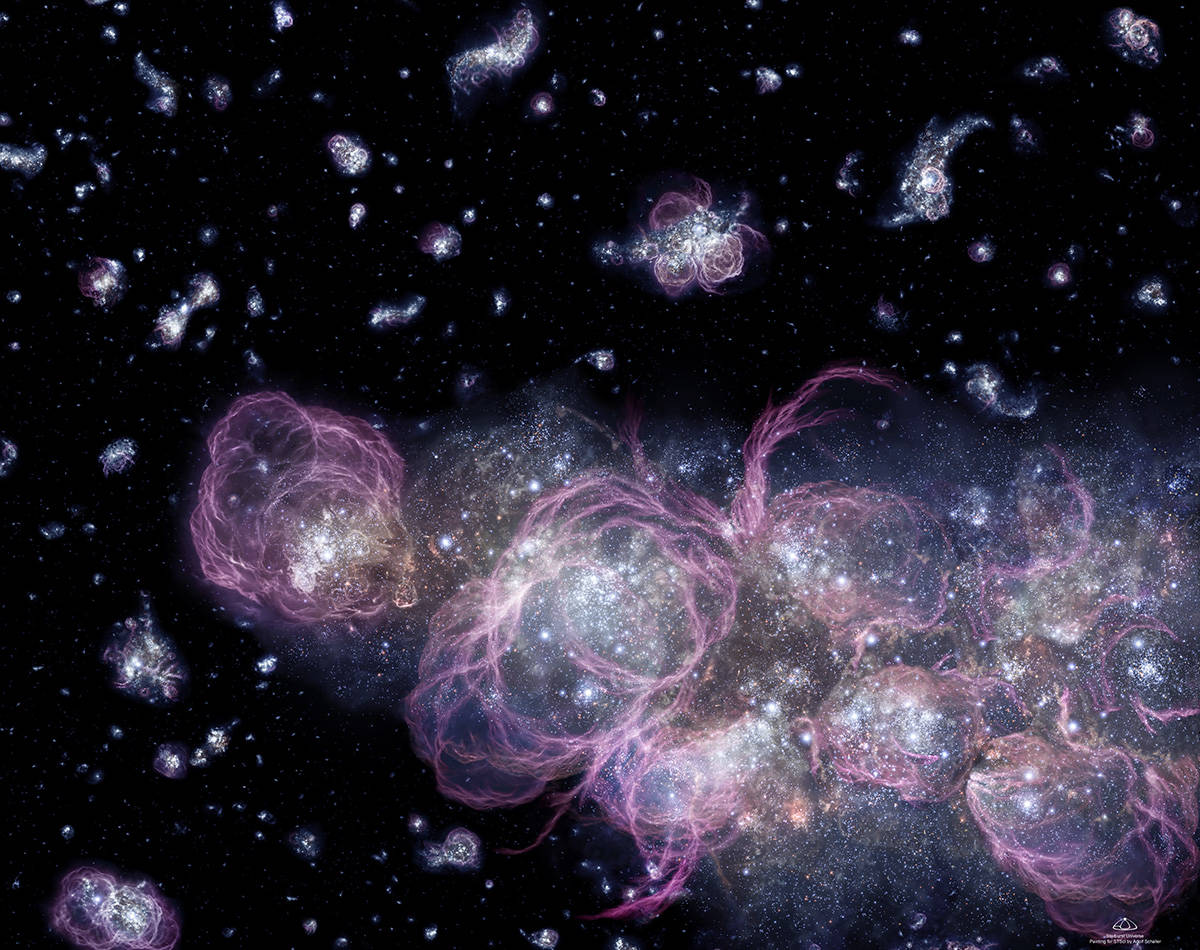
For one thing, Kroupa believes that such huge galaxy clusters shouldn’t even exist, never mind have had time to collide, at high redshifts. ΛCDM posits that structures should grow slowly, and Kroupa argues that it would be too slow for what our telescopes are showing us: massive galaxies and huge clusters in the early universe. More pertinently, it’s the dynamics of the cluster collisions themselves that give Kroupa hope. In particular, ΛCDM predicts that the velocities of the galaxies falling into the combined cluster’s gravitational well should be a lot lower than what is observed.
“Galaxy cluster collisions are in complete disagreement with ΛCDM while being in rather natural agreement with MOND,” says Kroupa. Despite Kroupa’s enthusiasm, McGaugh isn’t so sure. In fact, he thinks galaxy clusters are a real problem for both ΛCDM and MOND.
“It’s a mess,” he concedes. “For dark matter, the collision velocities are much too high. Dark-matter people have gone back and forth, arguing are the velocities too fast, or not? For MOND, it is that galaxy clusters show a mass discrepancy even after you apply MOND. Clusters concern me because I just don’t see a nice way out of that.”
A theory of everything?
Clusters and wide binaries can be debated ad infinitum until one side or the other admits defeat. But perhaps the most serious criticism levelled at MOND has been its outright lack of a workable cosmological model. It’s all well and good trying to replace dark matter with modified gravity in galaxies, but for the theory to ultimately be successful it must explain everything that dark matter can and more. This means it needs to be a rival to ΛCDM in explaining what we see in the cosmic microwave background (CMB) – the primordial microwave radiation that fills the universe.
The CMB is often characterized as the “fireball of the big bang”, but it’s more than that. Imprinted upon it in the form of subtle temperature variations from just 379,000 years after the Big Bang are what we call anisotropies, corresponding to regions of slightly higher or lower density formed by acoustic waves that reverberated through the primordial plasma. These are the seeds of structure formation in the universe. From these seeds grew the “cosmic web” – a network of filaments of matter along which galaxies grow and, where the filaments meet, large galaxy clusters.
MOND was devised to explain galaxy rotation curves by riffing on Newton, not Einstein. It took another 20 years for Bekenstein to come up with a relativistic model of MOND that could be applied to modern cosmology. Called Tensor–Vector–Scalar (TeVeS) gravity, it proved unpopular, struggling to explain the size of the third acoustic peak in the anisotropies that in the standard model is attributable to dark matter, as well as limitations in modelling gravitational lensing and gravitational waves.
Many people thought that the problem of a relativistic model of MOND was so difficult that it wasn’t possible. Then, in 2021 Constantinos Skordis and Tom Złośnik of the Czech Academy of Sciences proved everybody wrong. In their model, the duo introduced gravity-modifying vector and scalar fields that operate in the early universe to create gravitational effects that mimic dark matter, before evolving over time to resemble the regular MOND theory in the modern universe (Phys. Rev. Lett. 127 161302).
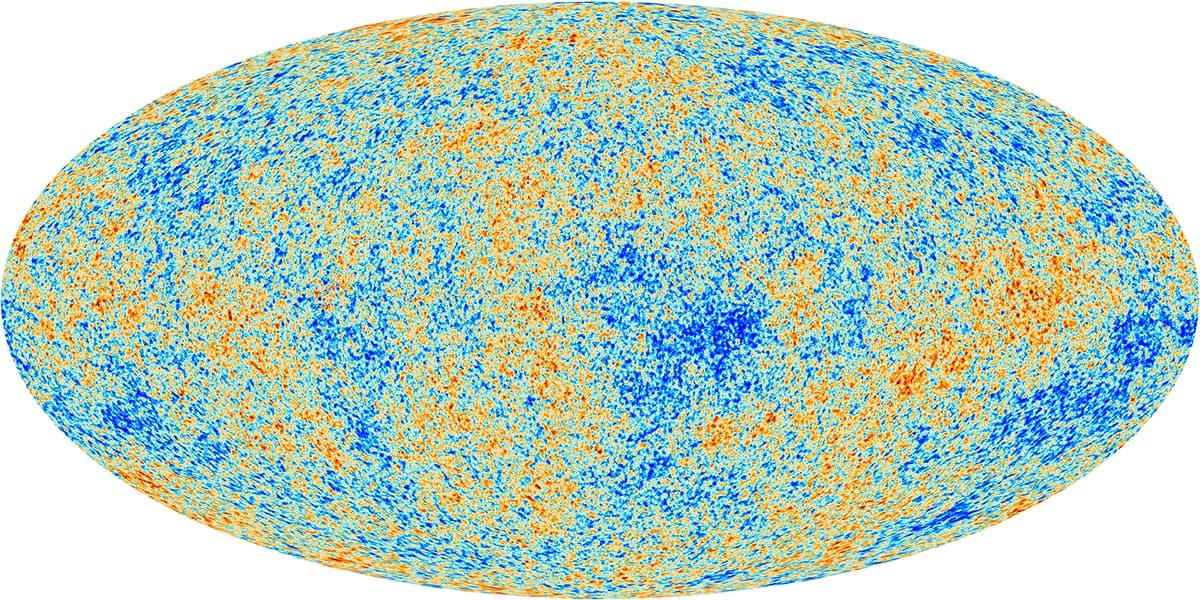
Given the torturous history of trying to develop a relativistic model of MOND, McGaugh believes it is a “remarkable accomplishment” to be able to write down such a theory that does fit the microwave background. The Skordis and Złośnik model is not perfect. Like TeVeS, it struggles to explain the amount of gravitational lensing we observe in the universe. Banik also highlights difficulties in the model, saying that “it got into difficulty in that it doesn’t provide a good explanation for galaxy clusters”.
Baker echoes these concerns. “While it was a good step forward for MOND to be able to do that,” he says, “I don’t think it was enough to bring MOND back into the mainstream. The reason being [Skordis and Złośnik] have added a lot of extra fields to it, a lot of bells and whistles, and it really loses elegance. It works with the CMB, but it seems very unnatural.”
Perhaps we’re putting undue weight onto the model’s shoulders. It could be viewed as just a beginning, a proof of concept. “Whether this is the final theory, or even down the right path, I don’t know,” says McGaugh. “But people have been saying that it can’t be done, and what Skordis and Złośnik have shown is that it can be done, and that’s an important step forward.”
MOND continues to fascinate, frustrate and foster disdain from dark matter’s disciples. There’s still a long way to go for the scientific community to consider it a heavyweight rival to ΛCDM, and it’s certainly hampered by having relatively few people working on it, meaning that progress is slow.
But the successes that this upstart theory has had shouldn’t be ignored, says McGaugh. If nothing else, it should keep astronomers working with the mainstream dark-matter model on their toes.
- In part two of Keith Cooper’s three-part series, he explores some of dark matter’s recent successes and the serious challenges it is also facing