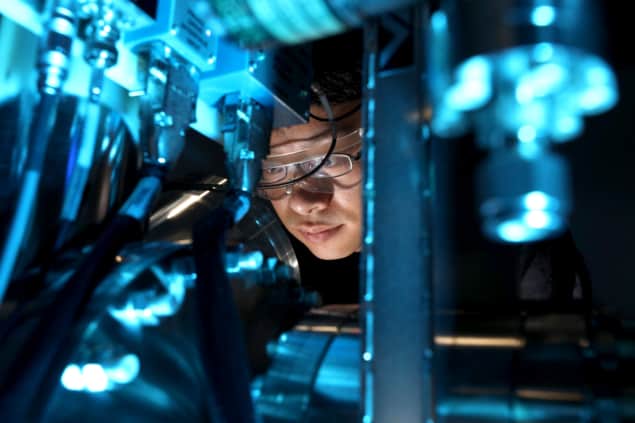
A new microscopy technique to measure “crackling noise” on the nanoscale could have a wide range of applications, from helping researchers better understand weak spots in metals to investigating biological structures such as kidney stones so they can be destroyed without the need for major surgery.
When a material is put under stress or strain, it triggers a series of atomic processes that can change a smooth motion such as a simple compression into a sequence of jerky ones. The result is a phenomenon known as crackling noise, which sounds rather like a creaking door but occurs in avalanche-like cascades that span many size scales and follow universal power laws.
“A typical case is when a compression produces cracks that do not progress in a simple line, but show complex patterns with lots of branches, like in a flash of lightning,” explains Ekhard Salje, a solid-state physicist at the University of Cambridge, UK, who co-led the new study with Jan Seidel of the University of New South Wales (UNSW) in Australia. “When there are many cracks, the material softens and may even disintegrate.”
Crackling noise was first studied in magnetic materials, where it is known as Barkhausen noise after the German physicist who discovered it in 1919. It is now used in materials science to investigate metal and alloys; in geophysics to study earthquakes; and in solid-state physics to develop memory devices in ferroic materials such as BaTiO3. “Each time the memory is activated, it initiates an avalanche,” explains Salje. “This avalanche helped researchers identify which materials were good for devices like memory switching.”
Observing the full spectrum of crackling noise
In the new work, members of the Cambridge-UNSW team used a technique based on atomic force microscopy (AFM) nanoindentation. They inserted the AFM probe extremely slowly – over a period of many hours – into the sample being studied. This slow insertion is important because if the probe moves too fast, even state-of-the-art electronic equipment will pick up too many overlapping signals, and thus see a continuous process rather than individual jerks, Salje says. This overlap makes it difficult to identify individual crackling noise signals.
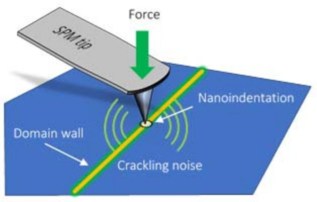
Thanks to their patient approach, the team was able to observe the full spectrum of crackling noise for the first time and relate it to specific forms of avalanche.
According to the researchers, the technique could have several uses. These include investigating special alloys for aircraft wings; studying corrosion in metals to identify weak spots where the metal breaks on the atomic scale; and testing the viability of new 3D printed materials. Salje says he is particularly interested in studying biological materials such as bones and teeth, which both emit crackling noise. Another important project, with the Addenbrooks hospital in Cambridge, is to study the crackling noise in kidney stones.
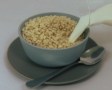
Snap, crackle and dam: puffed rice gives insights into rockfill and ice shelf collapse
“We can imagine building a tube with a needle at the end and testing kidney stones,” Salje explains. “This would help us to find out how to destroy them from the outside with having to resort to more invasive surgery.”
Seidel adds that he and his colleagues at the UNSW plan to use the technique to study topological defects in various functional materials. “We will also be looking at how to improve the measurement approach itself using an AFM system,” he reveals. “At the moment, I’m looking for a new PhD student to continue this work since the lead author of this work, which is published in Nature Communications, recently graduated from my group.”