
A polymer-based gel made by researchers in the US and inspired by the Venus flytrap plant can snap, jump and “reset” itself autonomously. The new self-propelled material might have applications in micron-sized robots and other devices that operate without batteries or motors.
“Many plants and animals, especially small ones, use special parts that act like springs and latches to help them move really fast, much faster than animals with muscles alone,” explains team leader Alfred Crosby, a professor of polymer science and engineering in the College of Natural Sciences at UMass Amherst. “The Venus flytraps are good examples of this kind of movement, as are grasshoppers and trap-jaw ants in the animal world.”
Snapping instabilities
The Venus flytrap plant works by regulating the way its turgor pressure – that is, the swelling produced as stored water pushes against a plant cell wall – is distributed through its leaves. Beyond a certain point, this swelling leads to a condition known as a snapping instability, where the tiny additional pressure of a fly’s footsteps is enough to cause the plant to snap shut. The plant then automatically regenerates its internal structures in readiness for its next meal.
Researchers have previously used these swelling-induced snapping instabilities to amplify the power of artificial motors, as they are good at converting the motor’s output into motion (think of pulling a rubber band and letting go). The problem is that so far, artificial gel-based devices have only been able to snap once, as they lack the internal self-regenerating mechanisms that makes repeated snaps possible in living structures like the Venus flytrap.
Transient deswelling
Crosby’s team got around this problem by harnessing an often-overlooked process called transient deswelling. They discovered the mechanism for this process by chance, when they observed a long, elastic strip of polymer gel moving as its internal liquid evaporated. Although most such movements were slow, every so often the researchers saw them speed up. “These faster movements were snap instabilities that continued to occur as the liquid evaporated further,” they explain. “Additional studies revealed that the shape of the material mattered and that the strips could reset themselves to continue their movements.”
To investigate the mechanisms behind this phenomenon, the researchers made a strip of material 0.2 mm thick, 5 mm wide and 60 mm long from poly(dimethylsiloxane), PDMS, and placed it in a solvent of n-hexane. They chose n-hexane because it has a high affinity for PDMS, allowing the material to fully swell up. Once the strip swelled, they placed it on a sheet of black poly(tetrafluoroethylene), PTFE. This polymer minimizes the effect of friction on the strip’s motion and prevents solvent from absorbing into the substrate.
Abrupt snaps
The researchers observed that during the first 90 seconds of deswelling, the strip buckled and transformed into different shapes in a seemingly random fashion. These complex buckling transitions included both smooth, simple bends and abrupt snaps.
To analyse how the snapping affected the strip’s movement, the researchers measured the change in the position of its apparent centre of mass and one endpoint (tip). They found that the centre of mass moved, on average, at 0.007 m/s, with a maximum speed or around 0.2 m/s. In contrast, the average speed of the tip was roughly 0.018 m/s, with a maximum of 1.1 m/s. This “extraordinarily” fast speed, which occurs at distinct moments and makes up only a few of the observed movements, must come from snapping, Crosby and colleagues say, since deformations caused by swelling or deswelling would only produce speeds of about 0.006 m/s or less.
Snapping structures perform complex tasks
The researchers, who detail their work in Nature Materials, also showed that the snapping motion could be used to perform tasks. “We used our understanding of the physics of the strip and made spherical shells from these structures,” Crosby tells Physics World. “These shells harnessed the snap instability to jump up a sloped substrate on their own as well as autonomously climb a ladder.”
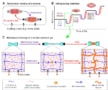
Hydrogel material flexes its muscles
The results demonstrate how materials can generate powerful movement by making use of interactions with their environment, such as through evaporation, he adds. “They are important for designing new robots, especially at small sizes where it’s difficult to have motors, batteries, or other energy sources.”
The UMass Amherst team says it’s now planning to extend its work on snapping strips and shells to create microscale bundles that act like synthetic muscles while generating power. “We will be able to more directly engineer these structures into microscale robots and other moving structures at small size scales,” says Crosby.