
Researchers in Spain have created a microfluidic cell culture platform that can generate highly realistic 2D cardiac tissue. The cost-effective, easy-to-use platform, based on electrospun nanofibres and electric stimulation, could prove invaluable to the cardiac research community for studies of heart disease and preclinical drug testing.
Cardiac muscle is a highly specialized tissue in which electrical signals are translated into synchronized fibre contractions that result in heart pumping. Creating realistic cardiac tissue in vitro is limited by difficulties in replicating the high level of organization and functional properties of adult cardiac tissues, in part due to the immaturity of the cells used in existing models.
To overcome these obstacles, the team – headed up at the Institute for Bioengineering of Catalonia and the University of Barcelona – used electrospinning to deposit nanofibrous scaffolds on thin coverslips. This approach enabled the researchers to tailor the fibre architecture (orientation, composition, thickness and density) to resemble that of the heart’s extracellular matrix and provide spatial cues to guide cardiac assembly in vitro.

“We have proposed a microfluidic device that allows us to reproduce some of the main characteristics of cardiac tissues,” says first author Adrián López-Canosa. “One of the most relevant is its high level of anisotropy, which means that the tissue is highly aligned in one particular direction. This was achieved by patterning the substrate of our device with aligned nanofibres.”
After patterning with electrospun nanofibres, the team bonded the coverslips to a silicone microfluidic device to create a 1300 x 8800 x 150 μm cell culture chamber. The device also incorporates two media channels and four holes to hold rod-shaped electrodes to stimulate the cells. This electrical stimulation is essential for maturation and organization of cardiac cells.
“It is unfeasible to obtain adequate cell numbers directly from adult donors, so the most common approach is to use cells from neonatal animal hearts or human pluripotent stem cells,” López-Canosa explains. “However, these cells are in a highly immature state, so implementing strategies to drive their maturation is paramount.”
Tissue growth
Optimal stimulation of cardiac cells requires a uniform electric field of 5 V/cm, 2 ms duration and 1 Hz frequency, to mimic the electrical impulses in murine native myocardium. López-Canosa and colleagues first developed a computational model of the platform’s electric field to determine whether it could meet these requirements.
They found that that the inexpensive rod-shaped electrodes could generate comparable electric fields to gold-standard planar electrodes with similar input voltages. Placing the electrodes tangential to the media channels and aligned with the cell chamber inlets gave the best trade-off between design simplicity and maximizing field strength in the chamber. Comparisons between the simulated voltage curve and experimental measurements revealed an excellent match between the two.
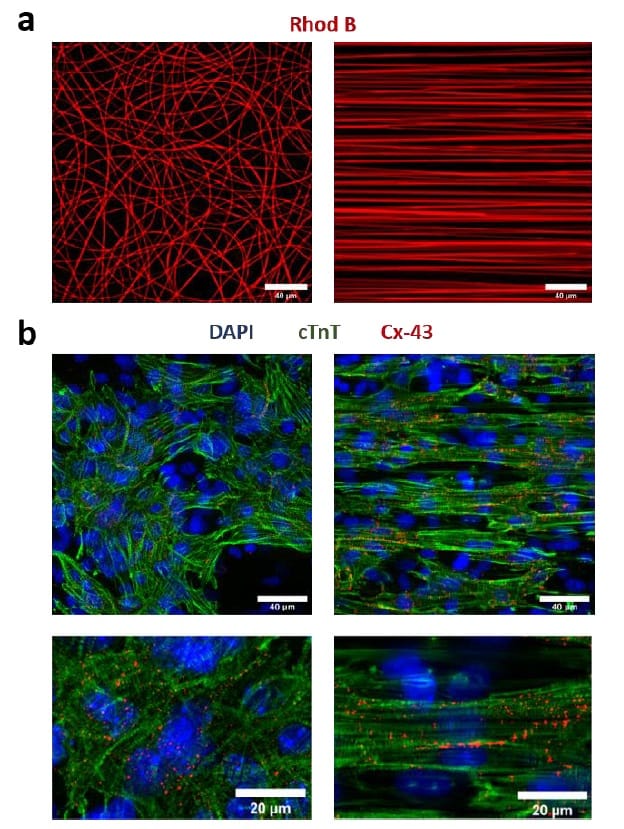
The researchers then validated the platform’s ability to grow cardiac tissue, using a co-culture of neonatal mouse cardiomyocytes (heart muscle cells) and cardiac fibroblasts (which produce connective tissue). They seeded the cells in the microfluidic device and evaluated the tissue after seven days in culture, including five days under electrical stimulation.
In platforms containing randomly deposited fibres, the distribution of cardiomyocyte contractile proteins was completely isotropic. A substrate of aligned fibres, on the other hand, yielded highly anisotropic cardiac tissues, with cells polarized in the direction of the nanofibres. The cardiomyocytes formed confluent cell monolayers that were capable of spontaneous contraction.
To assess the impact of electrical stimulation on the cardiac constructs, the team examined the development of gap junctions, which enable electrical signals to propagate across cardiac tissue and activate contraction. Immunofluorescence analyses revealed a moderate but significant increase in connexin-43 (the major gap junction protein in heart tissue) in stimulated tissue compared with unstimulated controls. They also observed increased expression of key cardiac genes related to contractile and conductive properties in the electrically stimulated samples.
The researchers conclude that their microfluidic system can generate highly biomimetic 2D cardiac tissue by combining topographical cues and electrical stimulation more simply and efficiently than previous platforms. Next, they plan to incorporate a system that generates oxygen gradients into the device.
“Moreover, we intend to make the platform 3D to achieve a higher degree of physiological mimicry,” says López-Canosa. “This will allow us to artificially mimic a myocardial infarction event, which would greatly benefit the field of cardiovascular research, as there is a clear lack of adequate models to study this disease.”
The researchers report their findings in Biofabrication.