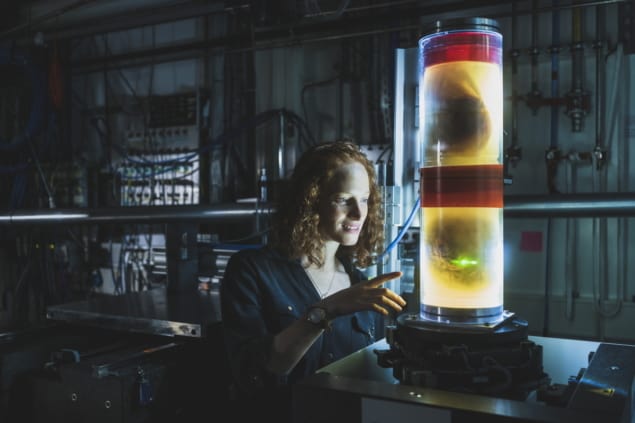
The ability to image human tissue at scales from an entire intact organ down to individual cells is key to advancing our understanding of health and disease. To meet this challenge, a European research collaboration has developed a new imaging technology known as hierarchical phase-contrast tomography (HiP-CT), using X-rays from the Extremely Brilliant Source (EBS) at the European Synchrotron Radiation Facility (ESRF).
The EBS is the first high-energy (6 GeV), fourth-generation synchrotron source. It provides the brightest source of X-rays in the world, with the spatial coherence required to resolve faint density contrasts at high resolution. Using the EBS, HiP-CT can perform non-destructive 3D scanning of an entire human organ and then zoom down to the cellular level.
“The ability to see organs across scales like this will really be revolutionary for medical imaging,” says first author Claire Walsh from University College London. “As we start to link our HiP-CT images to clinical images through AI techniques, we will – for the first time – be able to highly accurately validate ambiguous findings in clinical images. For understanding human anatomy, this is also a very exciting technique, being able to see tiny organ structures in 3D in their correct spatial context is key to understanding how our bodies are structured and therefore how they function.”
Walsh and colleagues have used the new synchrotron X-ray tomography technique to scan donated human organs, including lungs from a COVID-19 donor, reporting their findings in Nature Methods.
High-resolution scanning
Using the EBS test beamline, BM05, the researchers developed the specialized sample-preparation, scanning and reconstruction approaches required for HiP-CT. They designed a scanning geometry that reduces sample dose (to avoid tissue damage), optimizes the detector’s dynamic range, reduces artefacts and suppresses beam hardening.
For imaging, organs are fixed, partially dehydrated and stabilized in agar–ethanol in a jar. The team recorded reference scans of a jar of agar–ethanol mounted on top of the sample to provide a background that can be removed during image reconstruction. This process eliminates low-frequency background variations and enables extreme off-axis local reconstructions.
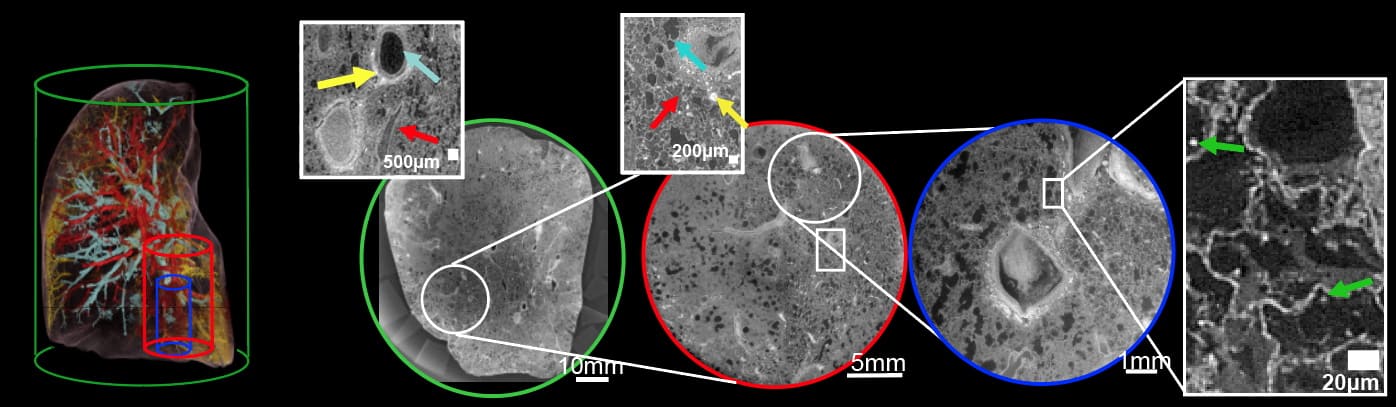
The HiP-CT scans are performed hierarchically, typically starting at 25 μm/voxel over the whole organ, then followed by magnified imaging of selected volumes-of-interest (VOIs) at 6.5 and 1.3–2.5 μm/voxel. They team assessed the performance of the HiP-CT technique by scanning an intact human lung. The estimated image resolutions were 72±3.4, 18.3±0.6 and 10.4±0.17 μm, for images recorded at 25, 6.5 and 2.5 μm/voxel, respectively.
To assess the consistency of scans at various depths and distances from the organ centre, the researchers analysed images of two high-resolution VOIs. They found minimal differences in mean intensity or image quality between the two, suggesting that HiP-CT can achieve high-resolution scanning in any region of the lung with consistent quality.
Next, they imaged five intact donated human organs – brain, lung, heart, kidney and spleen – performing HiP-CT at 25 μm/voxel to provide a structural overview of each organ, followed by multiple higher-resolution scans of selected VOIs. The 25 μm/voxel scans clearly identified macroscopic features such as individual lobules in the lung, for example, and the four chambers of the heart and associated coronary arteries.
The higher-resolution scans successfully visualized functional units in the organs, as well as imaging certain specialized cells. In the brain, for example, HiP-CT revealed layers of the cerebellum and individual Purkinje cells. Lung images showed the intralobular septa and septal veins, as well as terminal bronchi and bright cell-sized objects identified as pneumocytes and/or alveolar macrophages. Images of the heart showed bundles of cardiac muscle fibres comprising individual cardiomyocytes, while epithelial tubules were evident in the kidney, and red and white pulp was seen in the spleen.
COVID-19-related lung damage
The team also used HiP-CT to investigate structural changes in the lung tissue of a patient who died from COVID-19-related acute respiratory distress syndrome. Lung slices imaged at 25 μm/voxel contained high-intensity regions in the lung periphery, consistent with clinical radiology findings.

Scanning VOIs at 6 μm/voxel revealed heterogeneous parenchymal damage, with some secondary pulmonary lobules displaying greater deterioration than others. The team performed higher magnification images of a more affected lobule at 2 μm/per voxel. The scans revealed how severe COVID-19 infection remodels the finest blood vessels in the lungs, causing blood to shunt between the capillaries that supply oxygen to the entire body and those that feed the lung tissue itself. This cross-linking prevents the patient’s blood from being properly oxygenated – a process that was previously hypothesized but not proven.
“By combining our molecular methods with the HiP-CT multiscale imaging in lungs affected by COVID-19 pneumonia, we gained a new understanding of how shunting between blood vessels in a lung’s two vascular systems occurs in COVID-19 injured lungs, and the impact it has on oxygen levels in the circulatory system,” explains Danny Jonigk from Hannover Medical School.
The researchers emphasize that HiP-CT will continue to evolve alongside advances in synchrotron technology. Completion of a new ESRF beamline next year is anticipated to provide several key advances.
“The beam [will be] much larger and higher energy, hopefully enabling scanning of a whole torso at 20 micron voxels, while still being able to zoom locally to one micron. The increased beam size enables increased speed for the same sample size,” project lead Peter Lee tells Physics World. “We hope to form a hub to help us populate the Human Organ Atlas with a statistically significant number of organs in health and disease.”
- All image data from this study are publicly available in an online Human Organ Atlas.