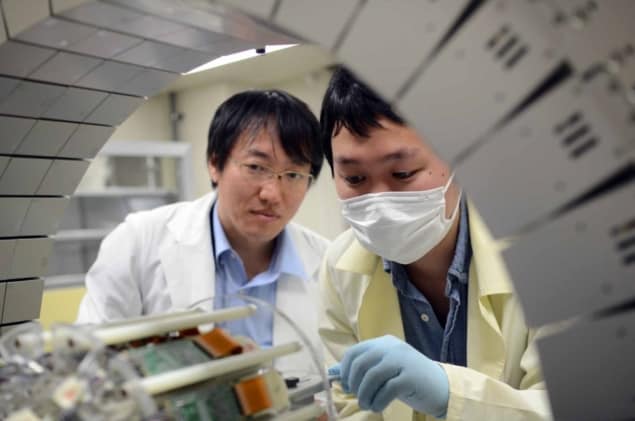
Compton imaging, originally developed by astronomers for detecting gamma ray sources, is now also under investigation for clinical imaging. In particular, a high-performance Compton camera could prove invaluable for applications within nuclear medicine and molecular imaging.
Unlike the established medical imaging technique of PET, which can only visualize positron emitters, Compton imaging has the potential to visualize a variety of gamma ray sources. To date, however, Compton image quality has not matched up to that of typical PET scans. To investigate its potential further, researchers at the National Institute of Radiological Sciences (NIRS) in Japan have created a combined whole gamma imaging (WGI) platform to directly compare the two modalities.
“Compton imaging has potential to provide better images than conventional SPECT and PET, in particular for radionuclides emitting high-energy gamma rays,” explains first author Hideaki Tashima. “We expect to explore new radionuclides that have never been used for nuclear medicine.”
A Compton camera incorporates two detectors that work in synchrony. For an individual gamma emission, Compton scattering occurs in the first detector (the scatterer) and photoelectric absorption in the second (the absorber). Both detectors record the interaction positions and corresponding deposited energies, enabling reconstruction of a Compton cone that indicates the emission point.
To create a WGI system that can perform both PET and Compton imaging, the researchers inserted a scatterer ring inside a whole-body depth-of-interaction PET scanner (the absorber ring). To enable small-animal imaging experiments, they remodelled a previous WGI prototype by halving the diameter of the scatterer ring. As the spatial resolution of Compton imaging reduces in proportion to the source–detector distance, this modification should also improve the resulting images.

System evaluation
Tashima and colleagues tested the WGI platform using 89Zr as the imaging source, as it decays via emission of a positron and a 909 keV gamma ray, enabling direct comparison of PET and Compton imaging. Alongside, they developed a 3D image reconstruction method incorporating detector response function (DRF) modelling, random correction and normalization. They report their findings in Physics in Medicine & Biology.
The researchers first evaluated the uniformity of the WGI prototype, by imaging a cylindrical phantom filled with 89Zr solution. When reconstructed without normalization, PET images exhibited ring artefacts and stripe patterns, while Compton images had higher coefficients of variation (COV) in the central region. The normalized PET and Compton images were of comparable quality, showing uniform radioactivity intensity throughout the phantom, and with COVs of 3.7% and 4.8% for PET and Compton images, respectively.
To assess the spatial resolution of the modalities, the researchers imaged a small rod phantom with clusters of cylindrical holes filled with 89Zr solution. The PET image clearly resolved the 2.2-mm diameter holes. The Compton image resolved the 3.0-mm holes in the peripheral region, with lower spatial resolution in the central region.
Finally, the team injected a mouse with 9.8 MBq 89Zr-oxalate and, one day later, used the WGI prototype to image the animal for 1 hr. The absorber ring (containing the PET detectors) had an axial length of 214 mm – enough to cover the whole animal. The PET image thus showed the entire mouse, revealing that 89Zr localized in its bones.

The scatterer ring was only 52 mm long, so the researchers positioned the animal’s torso inside the ring and its head and tail outside. Nevertheless, in Compton imaging mode, the WGI system could reconstruct the distribution outside the ring and create an image of almost the entire body. The Compton images agreed well with the PET images, clearly showing 89Zr in the mouse bones, although the image quality was better for regions inside the scatterer ring than those outside.
The researchers conclude that the WGI prototype could achieve Compton imaging with a quality approaching that of PET. They attribute this success to four key factors: the high energy of the gamma ray emitted from 89Zr, which improves the spatial resolution of Compton imaging; the DRF model used for image reconstruction, which further improved spatial resolution; the normalization step, essential for image uniformity; and the full-ring geometry of the WGI system.
Future work will focus on improving Compton imaging such that it outperforms PET. But the team’s ultimate goal is to unite the two techniques and implement a combined image reconstruction method. “Simultaneous measurement of different tracers with PET and Compton imaging can improve the efficiency of diagnosis,” explains Tashima. “In addition, reconstruction of a single tracer image by combining two types of signals can improve image quality through high sensitivity.”
“We are now exploring detector technologies for better energy resolution,” adds project leader Taiga Yamaya. “We are looking ahead to the realization of a clinical WGI system.”