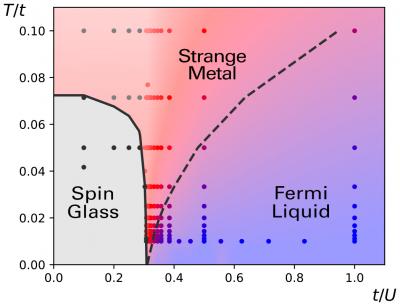
The first robust computational model of so-called “strange” metals has revealed that they are in fact a new state of matter. The model, developed by physicists from Cornell University and the Flatiron Institute, both in New York, could advance our understanding of other correlated quantum materials, such as high-temperature superconductors and even black holes.
Strange metals get their name from the peculiar behaviour of their electrons. Unlike electrons in ordinary metals, which travel freely with few interactions and little resistance, electrons in strange metals move sluggishly and in a restricted fashion. They also dissipate energy at the fastest possible rate allowed by the fundamental laws of quantum mechanics. In this sense, strange metals lie somewhere between metals and insulators, which have strongly-interacting electrons that occupy fixed positions.
But their strangeness doesn’t end there. For more than 30 years, researchers have puzzled over the fact that strange metals can become superconductors when cooled below a certain (relatively high) critical temperature. While models of this behaviour have existed for a while, they could not be accurately solved because the electrons are entangled, meaning that they cannot be treated as individual particles. What is more, any real material will have an enormous number of electrons, making exact solutions impossible.
Transition between an ordinary metal and an insulator
A team of researchers led by Eun-Ah Kim of Cornell’s Department of Physics have now solved this problem using a combination of two methods. First, they used a “quantum embedding” technique based on an approach developed in the 1990s by team member Antoine Georges of The Flatiron Institute’s Center for Computational Quantum Physics (CCQ). Here, the researchers performed detailed calculations on a few atoms in the system, rather than on the whole quantum system. They then used a quantum Monte Carlo algorithm, which relies on random sampling of the atoms, to solve the model of strange metals down to absolute zero.
A key feature of the resulting model, Kim explains, is that when the electrons’ kinetic energy is low, and their position therefore becomes more fixed, the system enters a so-called spin glass insulator state. In this state, random interactions between electron spins do not allow individual spins to point in the same direction as the spins of their neighbours, leading to “frustration” and a random alignment of spins.
Conversely, when kinetic energy is high, the electrons move freely, and the system enters a weakly-interacting state known as a Fermi liquid. In this state, large numbers of electrons can be modelled as non-interacting quasiparticles, each with an effective mass that exceeds that of a free electron. The Fermi-liquid model is good at predicting the properties of conventional metals – including the fact that, at low temperatures, the resistance of a conventional metal scales with the square of its temperature.
Kim points out that this scaling does not apply to strange metals. Instead, their electrical conductivity depends linearly on temperature, with the Planck and Boltzmann constants appearing as scaling factors. For this reason, strange metals are sometimes known as non-Fermi liquids or, alternatively, as Planckian metals.
A “reluctant” metal
By adjusting the ratio between the kinetic energy and interaction energy of the electrons in their model, the researchers were able to drive their model system to the verge of a transition between an ordinary metal and an interaction-driven insulator. At this point, strange metals appeared as a new state of matter, bordering these two known phases.

Strange metals become even stranger
“We found there is a whole region in the phase space that is exhibiting Planckian behaviour rather than belonging to either of the two phases that we’re transitioning between,” Kim says. In this quantum spin liquid state, the electrons are not completely locked down, but they are also not completely free either, she adds – making the material metallic, but only “reluctantly” so.
The new result, detailed in PNAS, could shed fresh light on the physics of high-temperature superconductors, which are related to Planckian metals in that their electrical resistance does not vary as expected with temperature either. More surprisingly, it could also have links to astrophysics. Like strange metals, black holes have properties (such as the length of time a black hole “resonates” after merging with another black hole) that depend only on temperature and the Planck and Boltzmann constants. “The fact that you find this same scaling across all these different systems is fascinating,” adds team member Olivier Parcollet of the CCQ.