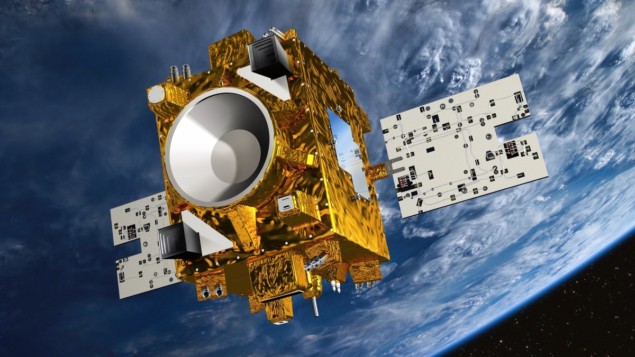
The equality of inertial and gravitational mass central to Einstein’s general theory of relativity has been confirmed at unprecedented sensitivities by the MICROSCOPE satellite. Having gathered several thousand orbits’ worth of accelerometer data from two masses in free fall around the Earth, the French mission has found no violation of the equivalence principle at the level of a few parts in a thousand trillion. Mission scientists say that better control of thermal and other noise could boost precision by a further factor of 100, so allowing tests of quantum-gravity theories.
Since it was published by Albert Einstein in 1915, the general theory of relativity has passed a host of experimental tests with flying colours – from the Sun’s deflection of starlight to the gravitational redshift of atomic clocks. But physicists regard the theory as incomplete because it is at odds with quantum mechanics, while the phenomena of dark matter and dark energy remain unexplained. Researchers would also like to unify gravity with the other three fundamental interactions of nature – electromagnetism and the strong and weak nuclear forces.
One way of hunting for new force carriers predicted by alternative theories of gravity is to subject the weak equivalence principle to ever more severe tests. This principle states that inertial and gravitational mass are equivalent. Therefore all objects, regardless of their mass and composition, should fall at the same rate in a gravitational field if not subject to other forces – such as variations in air pressure. (The strong version of the principle is more robust because it also considers the effects of self-gravitation, which becomes important for large objects.)
Eötvös ratio
Ever since Galileo Galilei, experimentalists have been probing the equivalence principle with increasing sensitivity. The metric used in modern tests is the Eötvös ratio, which compares the accelerations of two free-falling test masses and is zero if those accelerations are equal. In 2008, Eric Adelberger and colleagues at the University of Washington in Seattle, US, used a rotating torsion balance to obtain an Eötvös ratio of zero at the level of about 2 parts in 1013. While ten years later researchers at the Paris Observatory in France drew on nearly 50 years of laser-ranging data – looking for tiny variations in the Moon’s orbit of the Earth – and confirmed the equivalence principle with a precision of around 7×10-14.
The idea behind MICROSCOPE was to further improve precision by exploiting the virtues of being in Earth orbit – the fact that measurements can be carried out over long periods of time and without terrestrial interference such as seismic noise. The mission involved monitoring the relative acceleration of two concentric hollow cylinders made from different alloys – one consisting of titanium and aluminium and the other platinum and rhodium – as they travelled in continuous freefall. It did so by using electrodes to monitor any deviations in the cylinders’ movement and then applying a tiny voltage to set the cylinders straight – with variations in this applied voltage providing the signal for any violations of the equivalence principle.
The €140m MICROSCOPE mission was launched in 2016 by France’s CNES space agency in collaboration with researchers in Germany, the Netherlands and the UK. Placed into a nearly polar orbit with a period of around 1.5 h, the satellite yielded an initial data set – published in 2017 – from just 120 orbits. That resulted in a roughly order-of-magnitude improvement over the then record sensitivity – pushing the uncertainty in the zero value of the Eötvös ratio down to about 2 parts in 1014.
Much more data
The MICROSCOPE collaboration has now published the mission’s complete data set, acquired over the equivalent of five months within its 2.5-year mission lifetime (the satellite, still in orbit, will eventually burn up in the Earth’s atmosphere). Having at least an order of magnitude more data than five years ago, some of which came from a reference comparison between two cylinders made from the same material (platinum), the researchers have been able to reduce the uncertainty on the Eötvös ratio to some four parts in 1015 – and finding it still to be zero. That is not as precise as they were hoping – they wanted to reach one part in 1015 – but nevertheless represents a further improvement in precision by around a factor of five.
Scientists not involved with the mission welcome the new results, although Anna Nobili of the University of Pisa in Italy is sceptical that the precision is as high as stated. She points out that the biggest source of systematic error is thermal noise, resulting from temperature gradients set up by variations in direct and reflected sunlight reaching the spacecraft. She notes that with the satellite already in orbit, the only way to reduce the effects of this noise between the two data releases was to improve modelling of it. But she finds it “not fully convincing” that the modelling could have achieved the necessary reduction – a factor of six.
Nonetheless, Nobili reckons that MICROSCOPE shows the “huge potential of space” for very high precision tests of the equivalence principle. In particular, she argues that the mission demonstrates the importance of spinning a spacecraft at high rates to increase the frequency of any violation signal to levels where thermal noise is known to be lower. (She notes that the satellite was intended to spin at up to five times its orbital frequency but ended up spinning 17.5 times faster.)
Further noise reduction
MICROSCOPE collaboration member Joel Bergé of Université Paris Saclay says that he and his colleagues are now working on a larger follow-up mission called MICROSCOPE 2, which they have yet to propose to any space agency, but which could launch “in the second half of the 2030s”. He says that the new satellite would incorporate several changes to reduce noise, including the replacement of a gold wire used to remove unwanted charge from the test mass with a wireless system involving ultraviolet light-emitting diodes. Such changes, he claims, could reduce the measurement uncertainty to around one part in 1017.

The descent of mass
Clifford Will, a theorist at the University of Florida in the US, believes that the experience gained with the initial mission will give the MICROSCOPE researchers “a good foundation for moving to version 2.0”. He says that he is unable to judge the credibility of their projected 10-17 uncertainty but points out that scientists at Stanford University working on a proposed mission known as STEP argued that reaching that level of precision would necessitate the satellite being cooled down to cryogenic temperatures – something not envisaged for MICROSCOPE 2.
The research is described in papers published in Physical Review Letters and a special issue of Classical and Quantum Gravity.