
A new technique for reliably inserting single-ion impurities into a crystal and with a precision of just tens of nanometres could help in the development of quantum devices such as quantum computers or quantum simulators. The approach involves using a source of laser-cooled praseodymium ions to fabricate arrays of praseodymium colour centres in synthetic crystals of yttrium aluminium garnet (YAG). 50% of the impurities fluoresce – a success rate that is comparable to techniques that require ion energies three orders of magnitude higher.
Solid-state materials containing impurities such as nitrogen-vacancy colour centres or single rare-earth ions are a promising way to make scalable quantum information processors. The quantum states of these impurities can be tailored by laser and microwave pulses to perform quantum logic operations and the states read out by measuring their fluorescence. Precisely introducing ordered arrays of such impurities into crystals for scaling up quantum processors has proved difficult, however.
Deterministic single ion implantation with high placement precision
“Our technique allows for deterministic single ion implantation in a solid-state material with high placement precision,” explains Karin Groot-Berning of the Johannes Gutenberg University Mainz, who led this research effort. “The added advantage is that it can be applied to a large range of materials, doping ions and implantation energies. We believe it paves the way to the scalable fabrication of qubit arrays, such as those made of phosphorus qubits in ultra-pure silicon, for example. Being able to precisely place these arrays of single atoms in solids is an important step towards making quantum devices in which the arrays are the quantum register.”
Paul trap
Groot-Berning and colleagues began by loading and trapping a single praseodymium (Pr) ion and a single calcium (Ca) ion in a Paul trap. The Ca ion is laser-cooled so that the wave packet of the sympathetically cooled Pr ion also becomes very small (well below 100 nm in size), she explains.
The researchers extract both ions by applying an electric field. They “blank” away the Ca ion, but steer the Pr ion into a lens and focus it down to a spot size of about 30 nm.
“The Pr ion then hits the surface of a YAG crystal, which is a synthetic crystal commonly employed as a lasing medium, with a speed of 73 km/s and it enters the material to a depth of about 6 nm,” says Groot-Berning. “We repeat this procedure to inject a succession of Pr ions into the crystal.”
Forming a colour centre
“The crystal can be moved with a piezo-translation state and we can implant any pattern,” she tells Physics World. “We perform the measurements in an ultrahigh vacuum apparatus in Mainz, where we can trap, cool and extract the ions. Finally, we take the YAG out of the apparatus and send the sample to our colleagues at the Physical Institute of the University of Stuttgart. Here, they flash-heat the crystal to 1200°C such that the Pr ions replace the yttrium ions in the crystal lattice, thus forming a colour centre.”
The researchers then use a set of lasers to excite the array so that it emits photons, which they can detect with a confocal microscope. They found that they could control the position of the Pr ions to a precision of 34 nm and that up to 50% of the colour centres fluoresced.
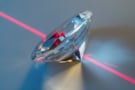
Quantum Darwinism spotted in diamond spins
Precision could be improved further
This precision could be improved further, because it is currently limited by imperfect cooling and mechanical vibrations, says Groot-Berning. “Indeed, we have already started to work on this problem and improve the mechanical stability of our set up in a second-generation implanter.”
The team, reporting its work in Physical Review Letters, says that it now plans to use its technique to implant phosphorus ions into silicon to form arrays of quantum bits. “We also plan to investigate implanting bismuth ions, for coupling their nuclear spins to superconducting quantum bit devices, and cerium ions into the YAG crystal, because this rare earth ion allows for super-resolution microscopy (STED),” reveals Groot-Berning. “This will allow us to fabricate qubit devices using these ions and detect them with even better resolution – down to a few nm.
“Our current placement accuracy is already sufficient for fabricating quantum devices, however,” she stresses.